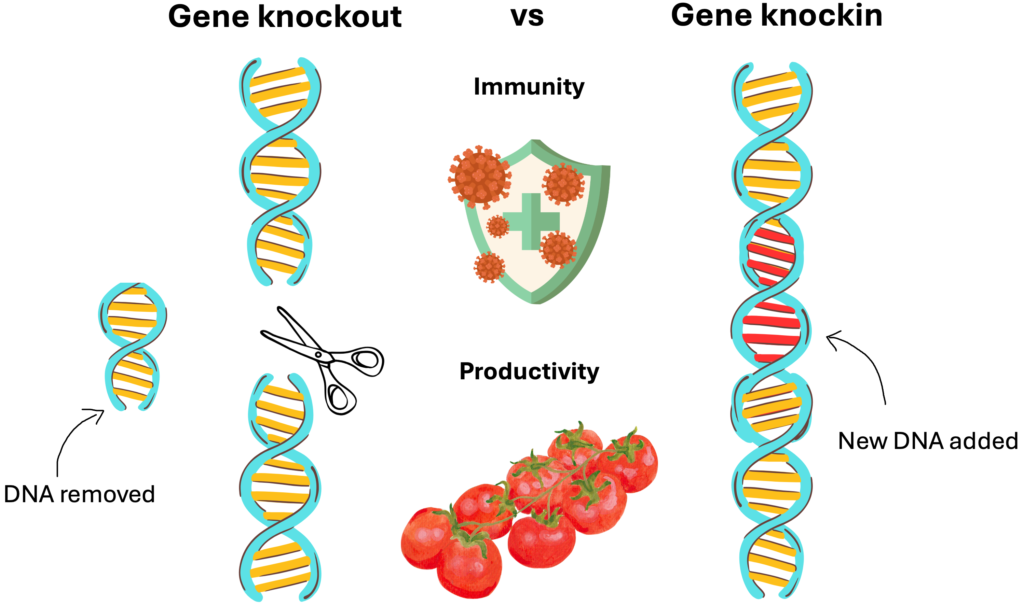
Image: Cartoon showing contrasting approaches of gene knockout and gene knockin to improve crop immunity and productivity.
CRISPR technology has ushered in a new era of genetic research, allowing scientists to edit DNA with precision. Whether you’re aiming to improve crop yields, enhance disease resistance, or introduce entirely new traits, CRISPR’s gene knockout and knockin techniques offer incredible possibilities. In this blog, we’ll explore the differences between gene knockout and knockin—two powerful methods that are transforming plant science—and guide you through the step-by-step pipeline of how they work.
What’s the Difference? Gene Knockout vs. Knockin
Before we delve into the pipeline, let’s clarify the fundamental difference between these two gene-editing approaches:
- Gene Knockout is like hitting the “delete” button. In a knockout, you deactivate or entirely remove the function of a specific gene. For instance, if your tomato plant has a gene that limits branching, knocking it out might encourage the plant to grow more branches—and ultimately produce more fruit.
- Gene Knockin is more like a “find and replace” function in a word processor. Instead of just deleting a gene, knockin involves replacing it with an improved or synthetic version. Imagine replacing a gene in your tomato plant with one from a wild variety that naturally produces more fruit. Knockin allows for this precise kind of gene swapping.
Step-by-Step Pipeline: How Knockout and Knockin Work
Now that we understand the basic concept, let’s walk through the pipeline of how gene knockout and knockin are executed using CRISPR.
1. Choosing the Right Cas Protein
CRISPR technology relies on Cas proteins, the “scissors” that cut DNA. Choosing the correct Cas protein is crucial for the type of edit you want to make:
- Cas9: This is the go-to Cas protein for most gene-editing tasks. It targets a specific DNA sequence (NGG) found in protein-coding regions of genes, making it ideal for gene knockouts.
- Cas12: For more specialized tasks, especially those involving regulatory regions (non-coding parts of DNA), Cas12 is the better choice. It recognizes the sequence TTTN, giving scientists the ability to cut and edit DNA in different areas than Cas9.
2. Designing the Guide RNA
Once the Cas protein is selected, the next step is to design a guide RNA (gRNA). This gRNA acts as the GPS for the Cas protein, guiding it to the exact location on the DNA where the edit needs to be made. A typical guide RNA is about 18-24 bases long and is highly specific to the target gene to avoid unintended cuts in other areas of the genome.
Web-based tools such as CRISPOR (http://crispor.gi.ucsc.edu/) and CRISPR-P 2.0 (http://crispr.hzau.edu.cn/cgi-bin/CRISPR2/CRISPR) make designing guide RNAs relatively simple. These tools help ensure that the gRNA is tailored precisely to your target gene, whether you’re aiming for a knockout or a knockin.
3. Building the Plasmid
Next, the gRNA, Cas protein, and any additional components—such as fluorescent markers or antibiotic resistance genes—are inserted into a plasmid. A plasmid is a small, circular DNA molecule that acts like a delivery vehicle, carrying all the necessary tools into the plant cells.
For knockin, the plasmid also carries the synthetic version of the gene you want to insert, known as a donor template. This template will be used to replace the target gene after the Cas protein makes the cut.
4. Delivering the Plasmid
Once the plasmid is built, it needs to be delivered into the plant cells. Here’s where nature lends a hand. Scientists use a bacterium called Agrobacterium, which naturally transfers DNA to plant cells. This bacterium acts as a shuttle, delivering the plasmid into the plant’s genome.
This can be done in two main ways:
- Floral dip: Immature flowers are directly treated with Agrobacterium to introduce the plasmid.
- Callus tissue culture: Plant tissues are grown in a petri dish and treated with Agrobacterium to deliver the plasmid.
5. Editing the DNA
Once inside the plant, the plasmid gets to work. The guide RNA directs the Cas protein to the target gene, where it makes a precise cut in the DNA:
- Gene Knockout: After the cut is made, the plant’s natural repair mechanisms attempt to fix the break, often leading to small mutations that disable the gene—effectively knocking it out.
- Gene Knockin: For knockin, the synthetic gene (provided by the donor template on the plasmid) is inserted into the DNA at the cut site. This allows the new gene to take the place of the old one, like swapping out a puzzle piece.
6. Selecting the Edited Plants
After the gene-editing process is complete, it’s time to identify which plants successfully incorporated the desired changes. To make this process easier, the plasmid contains fluorescent proteins and antibiotic resistance genes.
- Fluorescent marker: Under a microscope, seeds that have successfully taken up the plasmid will glow due to the fluorescent marker.
- Antibiotic resistance: Seeds are grown in an antibiotic-containing medium. Only those with the correct gene edits (either knockout or knockin) will survive in this environment, making it easy to identify successful edits.
7. Verifying the Edits
The final step is to confirm that the gene editing has been successful. This is done through DNA sequencing, which verifies that the target gene was either knocked out or replaced with the synthetic gene. Once confirmed, the new genetically edited plants are ready to be grown, bringing us closer to more productive, resilient crops.
The Power of CRISPR: A Future Full of Possibilities
Gene knockout and knockin are powerful tools that allow us to make precise genetic changes in plants, offering exciting opportunities in agriculture. Whether you’re looking to enhance crop yields, improve disease resistance, or develop plants that can withstand the pressures of climate change, these techniques hold the key.
CRISPR is not just about deleting or replacing genes—it’s about pushing the boundaries of what’s possible in plant science. With these technologies, we can unlock new traits, overcome agricultural challenges, and shape the future of farming.
Stay tuned for more insights into how CRISPR is transforming plant biology and changing the way we think about crop development. Whether you’re a scientist or a gardening enthusiast, CRISPR opens up a world of possibilities for the plants of tomorrow.
In case you missed my first blog on Gene Knockin technology click here.
References
1. Zhu, H., Li, C. & Gao, C. Applications of CRISPR–Cas in agriculture and plant biotechnology. Nat Rev Mol Cell Biol 21, 661–677 (2020). https://doi.org/10.1038/s41580-020-00288-9.
2. Cardi, T et al. CRISPR/Cas-mediated plant genome editing: outstanding challenges a decade after implementation. Trends in Plant Science, 28, 1144 – 1165 (2023).
Pingback: CRISPR Gene Knockin: Revolutionizing Plant Science – plantsdecoded.com